How do marine invertebrates detect sounds?
There is evidence to suggest that invertebrates can detect both water-borne and substrate-borne particle motion (vibration). Water-borne particle motion is detected as movement in the surrounding aquatic medium, whereas substrate-borne vibration is detected as particle motion below the seafloor. Semi-terrestrial crabs[1]Popper, A. N., Salmon, M., & Horch, K. W. (2001). Acoustic detection and communication by decapod crustaceans. Journal of Comparative Physiology A: Sensory, Neural, and Behavioral Physiology, 187(2), 83–89. https://doi.org/10.1007/s003590100184. and seafloor-dwelling marine invertebrates, such as mussels [2]Roberts, L., Cheesman, S., Breithaupt, T., & Elliott, M. (2015). Sensitivity of the mussel Mytilus edulis to substrate‑borne vibration in relation to anthropogenically generated noise. Marine Ecology Progress Series, 538, 185–195. https://doi.org/10.3354/meps11468. and hermit crabs[3]Roberts, L., Cheesman, S., Elliott, M., & Breithaupt, T. (2016). Sensitivity of Pagurus bernhardus (L.) to substrate-borne vibration and anthropogenic noise. Journal of Experimental Marine Biology and Ecology, 474, 185–194. https://doi.org/10.1016/j.jembe.2015.09.014., have been shown to respond to substrate-borne vibration.
Marine invertebrates may detect particle motion using external sensory hairs, internal statocysts, and chordotonal organs on body appendages[4]Budelmann, B. U. (1992). Hearing in Nonarthropod Invertebrates. In D. B. Webster, A. N. Popper, & R. R. Fay (Eds.), The Evolutionary Biology of Hearing (pp. 141–155). Springer New York. https://doi.org/10.1007/978-1-4612-2784-7_10.. Although some marine invertebrates that produce sound in air have specialized sensory organs that can detect pressure changes in air, most groups do not have organs for detecting water pressure and also lack the air-filled spaces and compressible tissues required for pressure detection.
Sensory hairs
Marine crustaceans (e.g., lobster, crabs, and shrimp) have an extensive array of sensory hairs covering their bodies, which have been shown to function in a similar way to the fish lateral line[5]Breithaupt, Th., & Tautz, J. (1988). Vibration sensitivity of the crayfish statocyst. Naturwissenschaften, 75(6), 310–312. https://doi.org/10.1007/BF00367325.[6]Wiese, K. (1976). Mechanoreceptors for near-field water displacements in crayfish. Journal of Neurophysiology, 39(4), 816–833. https://doi.org/10.1152/jn.1976.39.4.816.. Water- or substrate-borne vibrations associated with changes in acceleration, hydrodynamic flow, and/or sound may stimulate these sensory hairs. Detecting these vibrations may help animals sense the movements of other nearby organisms and their surroundings. Researchers found sensory hairs located on the claws of the Australian freshwater crayfish to be most sensitive to water-borne particle motion at frequencies between 150 and 300Hz[7]Tautz, J., & Sandeman, D. C. (1980). The detection of waterborne vibration by sensory hairs on the chelae of the crayfish. Journal of Experimental Biology, 88(1), 351–356. https://doi.org/10.1242/jeb.88.1.351.. Sensory hairs found on different body parts of the American lobster can detect low frequency, underwater sounds from 80 to 250 Hz[8]Jézéquel, Y., Jones, I. T., Bonnel, J., Chauvaud, L., Atema, J., & Mooney, T. A. (2021). Sound detection by the American lobster ( Homarus americanus ). Journal of Experimental Biology, 224(6), jeb240747. https://doi.org/10.1242/jeb.240747.. Other invertebrate groups, such as coelenterates, ctenophores, annelids and flatworms are known to respond to water movement or vibrations using sensory hair cells or other epidermal structures for detection[9]Budelmann, B. U. (1992). Hearing in Crustacea. In D. B. Webster, A. N. Popper, & R. R. Fay (Eds.), The Evolutionary Biology of Hearing (pp. 131–139). Springer New York. https://doi.org/10.1007/978-1-4612-2784-7_9..
The statocyst
The statocyst is a complex sensory organ found in most marine invertebrates, consisting of a dense mass (the statolith) inside a fluid filled chamber. It primarily provides orientation cues that allow an animal to maintain its position in the water column[10]Cohen, M. J. (1955). The function of receptors in the statocyst of the lobster Homarus americanus. The Journal of Physiology, 130(1), 9–34. https://doi.org/10.1113/jphysiol.1955.sp005389.[11]Cohen, M. J., & Dijkgraaf, S. (1961). Mechanoreception. In The Physiology of Crustacea (2nd ed., pp. 65–108). Academic Press.[12]Lovell, J. M., Moate, R. M., Christiansen, L., & Findlay, M. M. (2006). The relationship between body size and evoked potentials from the statocysts of the prawn Palaemon serratus. Journal of Experimental Biology, 209(13), 2480–2485. https://doi.org/10.1242/jeb.02211.. In some animals, the statocyst may also function to detect the low frequency, particle motion component of sound.
In cephalopods, studies with electrodes placed near the statocysts showed longfin squids had a neural response to sounds between 30 and 500 Hz. The response was greatest at frequencies between 100 and 200 Hz. When the sensory function of a longfin squid statocyst was surgically altered, sensitivity to acoustic stimuli was eliminated[13]Mooney, T. A., Hanlon, R. T., Christensen-Dalsgaard, J., Madsen, P. T., Ketten, D. R., & Nachtigall, P. E. (2010). Sound detection by the longfin squid (Loligo pealeii) studied with auditory evoked potentials: Sensitivity to low-frequency particle motion and not pressure. Journal of Experimental Biology, 213(21), 3748–3759. https://doi.org/10.1242/jeb.048348.. Similar studies on oval squid and common octopus showed neurological responses to sounds from 400-1500 Hz and 400-1000 Hz, respectively[14]Hu, M. Y., Yan, H. Y., Chung, W.-S., Shiao, J.-C., & Hwang, P.-P. (2009). Acoustically evoked potentials in two cephalopods inferred using the auditory brainstem response (ABR) approach. Comparative Biochemistry and Physiology Part A: Molecular & Integrative Physiology, 153(3), 278–283. https://doi.org/10.1016/j.cbpa.2009.02.040..
3D rotation of a cuttlefish head
3D rotation of a cuttlefish (Sepia officialis) head showing the statocysts (the two small, bulbous objects in the center of the frame). Video credit: Ketten Laboratory and the Computerized Scanning and Imaging facility (CSI) https://csi.whoi.edu of Woods Hole Oceanographic Institution.
Many marine crustaceans also possess statocysts, usually located on their first antennae or in the abdomen[15]Cohen, M. J., & Dijkgraaf, S. (1961). Mechanoreception. In The Physiology of Crustacea (2nd ed., pp. 65–108). Academic Press.. Researchers found the statocyst of the common prawn to respond to sounds ranging from 100-3000 Hz with electrodes positioned on the antennules[16]Lovell, J. M., Findlay, M. M., Moate, R. M., & Yan, H. Y. (2005). The hearing abilities of the prawn Palaemon serratus. Comparative Biochemistry and Physiology Part A: Molecular & Integrative Physiology, 140(1), 89–100. https://doi.org/10.1016/j.cbpb.2004.11.003.. The statocysts of snapping shrimp were found to be responsive to stimuli ranging from 40 to 1500 Hz[17]Dinh, J. P., & Radford, C. (2021). Acoustic particle motion detection in the snapping shrimp (Alpheus richardsoni). Journal of Comparative Physiology A, 207(5), 641–655. https://doi.org/10.1007/s00359-021-01503-4..
Not all marine crustaceans that have statocysts use them for sound detection. Researchers found that removing the statocyst of the American lobster did not eliminate their neural response to acoustic stimuli. However, immobilizing body surface sensory hairs did eliminate the response[18]Jézéquel, Y., Jones, I. T., Bonnel, J., Chauvaud, L., Atema, J., & Mooney, T. A. (2021). Sound detection by the American lobster ( Homarus americanus ). Journal of Experimental Biology, 224(6), jeb240747. https://doi.org/10.1242/jeb.240747..
There are indications that non-cephalopod mollusks such as the Pacific oyster, common cockle, swift scallop, and various clam species are sensitive to sound or vibrational exposures. Scientists hypothesized that bivalves such as these detect sounds and vibrations either with the statocyst or external body hairs[19]Budelmann, B. U. (1992). Hearing in Crustacea. In D. B. Webster, A. N. Popper, & R. R. Fay (Eds.), The Evolutionary Biology of Hearing (pp. 131–139). Springer New York. https://doi.org/10.1007/978-1-4612-2784-7_9.[20]Lacourse, J. R., & Northrop, R. B. (1978). A preliminary study of mechanoreceptors within the anterior byssus retractor muscle of Mytilus edulis L. The Biological Bulletin, 155(1), 161–168. https://doi.org/10.2307/1540873.; but there are few studies investigating sound and substrate vibration detection in mollusks. Pacific oysters suspended in a mesh bag in an experimental flume exhibited valve-closing behaviors in response to playback sounds in the frequency range of 10 – 1000 Hz[21]Charifi, M., Sow, M., Ciret, P., Benomar, S., & Massabuau, J.-C. (2017). The sense of hearing in the Pacific oyster, Magallana gigas. PLOS ONE, 12(10), e0185353. https://doi.org/10.1371/journal.pone.0185353.. Laboratory experiments using a shaker-table showed blue mussels responded to substrate-borne vibrations between 5 and 400 Hz, with sensitivity being relatively constant across all frequencies before reaching a decrease in sensitivity at 200 Hz[22]Roberts, L., Cheesman, S., Breithaupt, T., & Elliott, M. (2015). Sensitivity of the mussel Mytilus edulis to substrate‑borne vibration in relation to anthropogenically generated noise. Marine Ecology Progress Series, 538, 185–195. https://doi.org/10.3354/meps11468..
Chordotonal organs
Chordotonal organs, found in semi-terrestrial crustaceans, are generally associated with the joints of flexible appendages (e.g. walking legs and/or antennae) and are connected to the central nervous system. Movements or vibrations transmitted through the substrate can stimulate the chordotonal organs. Specialized Barth’s myochordotonal organs (Barth’s MCO) are found in fiddler and ghost crabs[23]Horch, K. (1971). An organ for hearing and vibration sense in the ghost crab Ocypode. Zeitschrift Fur Vergleichende Physiologie, 73(1), 1–21. https://doi.org/10.1007/BF00297698.[24]Salmon, M., Horch, K., & Hyatt, G. W. (1977). Barth’s myochordotonal organ as a receptor for auditory and vibrational stimuli in fiddler crabs (Uca pugilator and U. minax). Marine Behaviour and Physiology, 4(3), 187–194. https://doi.org/10.1080/10236247709386951.. Externally, the organ can be identified as a distinct, thin-walled “window” in the exoskeleton on each leg. In ghost crabs, this sensory organ is receptive to both substrate-borne and airborne sounds[25]Horch, K. (1971). An organ for hearing and vibration sense in the ghost crab Ocypode. Zeitschrift Fur Vergleichende Physiologie, 73(1), 1–21. https://doi.org/10.1007/BF00297698., while in fiddler crabs, it responds primarily to substrate-borne vibrations[26]Salmon, M., Horch, K., & Hyatt, G. W. (1977). Barth’s myochordotonal organ as a receptor for auditory and vibrational stimuli in fiddler crabs (Uca pugilator and U. minax). Marine Behaviour and Physiology, 4(3), 187–194. https://doi.org/10.1080/10236247709386951.. Chordotonal organs typically detect sounds and substrate-borne vibrations with frequencies greater than 300 Hz, which could allow them to detect sound above background noise in their tidal habitats[27]Horch, K. (1971). An organ for hearing and vibration sense in the ghost crab Ocypode. Zeitschrift Fur Vergleichende Physiologie, 73(1), 1–21. https://doi.org/10.1007/BF00297698.[28]Salmon, M., Horch, K., & Hyatt, G. W. (1977). Barth’s myochordotonal organ as a receptor for auditory and vibrational stimuli in fiddler crabs (Uca pugilator and U. minax). Marine Behaviour and Physiology, 4(3), 187–194. https://doi.org/10.1080/10236247709386951.[29]Popper, A. N., Salmon, M., & Horch, K. W. (2001). Acoustic detection and communication by decapod crustaceans. Journal of Comparative Physiology A: Sensory, Neural, and Behavioral Physiology, 187(2), 83–89. https://doi.org/10.1007/s003590100184.. Interestingly, if a crab were to lose a leg (to escape a predator or during a fight), the animal’s acoustic sensitivity would be minimally reduced[30]Horch, K. (1971). An organ for hearing and vibration sense in the ghost crab Ocypode. Zeitschrift Fur Vergleichende Physiologie, 73(1), 1–21. https://doi.org/10.1007/BF00297698.[31]Popper, A. N., Salmon, M., & Horch, K. W. (2001). Acoustic detection and communication by decapod crustaceans. Journal of Comparative Physiology A: Sensory, Neural, and Behavioral Physiology, 187(2), 83–89. https://doi.org/10.1007/s003590100184. because all walking legs contain the sensory organ, and hence, a crab would still be able to detect vibrations through its other walking legs.
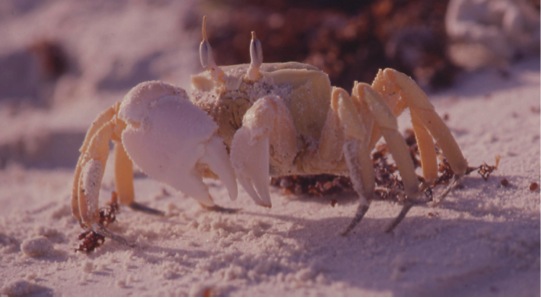
Ocypode saratan, a species of semi-terrestrial ghost grab. These crabs possess Barth’s myochordotonal organs, specialized sensory organs in the walking legs that detect substrate-borne and airborne sounds. Image courtesy of David Clayton, Sultan Qaboos University (Oman).
Additional Links on DOSITS
- Science of Sound > Advanced Topics > What is intensity?
- Animals and Sound > Advanced Topics > What components of sound are used for hearing?
- Animals and Sound > How do marine invertebrates produce sound?
- Audio Gallery > Snapping shrimp
Additional Resources
- Watson, W. (n.d.) Lobster Bioacoustics Research. University of New Hampshire. Retrieved from http://lobsters.unh.edu/bioacoustic/bioacoustic.html
References
- Cragg, S. M., & Nott, J. A. (1977). The ultrastructure of the statocysts in the pediveliger larvae of Pecten maximus (L.) (Bivalvia). Journal of Experimental Marine Biology and Ecology, 27(1), 23–36. https://doi.org/10.1016/0022-0981(77)90051-X
- Derby, C. D. (1982). Structure and function of cuticular sensilla of the lobster Homarus Americanus. Journal of Crustacean Biology, 2(1), 1–21. https://doi.org/10.2307/1548106
- Factor, J. R. (Ed.). (1995). Biology of the Lobster Homarus americanus. San Diego: Academic Press.
- Kaifu, K., Segawa, S., & Tsuchiya, K. (2007). Behavioral responses to underwater sound in the small benthic octopus Octopus ocellatus. The Journal of the Marine Acoustics Society of Japan, 34(4), 266–273. https://doi.org/10.3135/jmasj.34.266
- Kaifu, K., Akamatsu, T., & Segawa, S. (2008). Underwater sound detection by cephalopod statocyst. Fisheries Science, 74(4), 781–786. https://doi.org/10.1111/j.1444-2906.2008.01589.x
- Mooney, T. A., Hanlon, R., Madsen, P. T., Christensen-Dalsgaard, J., Ketten, D. R., & Nachtigall, P. E. (2012). Potential for Sound Sensitivity in Cephalopods. In A. N. Popper & A. Hawkins (Eds.), The Effects of Noise on Aquatic Life (Vol. 730, pp. 125–128). New York, NY: Springer New York. https://doi.org/10.1007/978-1-4419-7311-5_28
- Packard, A., Karlsen, H. E., & Sand, O. (1990). Low frequency hearing in cephalopods. Journal of Comparative Physiology A, 166(4). https://doi.org/10.1007/BF00192020
- Salmon, M. (1983). Acoustic “calling” by fiddler and ghost crabs. In Papers from the Conference on the Biology and Evolution of Crustacea. Australian Museum Memoir 18 (pp. 63–76). Sydney, New South Wales, Australia: Australian Museum.
- Taylor, J. R. A., & Patek, S. N. (2010). Crustacean seismic communication: Heard by not present? In C. E. O’Connell-Rodwell (Ed.), The Use of Vibrations in Communication: Properties, Mechanisms and Function across Taxa (pp. 9–23).
Cited References
⇡1, ⇡29, ⇡31 | Popper, A. N., Salmon, M., & Horch, K. W. (2001). Acoustic detection and communication by decapod crustaceans. Journal of Comparative Physiology A: Sensory, Neural, and Behavioral Physiology, 187(2), 83–89. https://doi.org/10.1007/s003590100184. |
---|---|
⇡2, ⇡22 | Roberts, L., Cheesman, S., Breithaupt, T., & Elliott, M. (2015). Sensitivity of the mussel Mytilus edulis to substrate‑borne vibration in relation to anthropogenically generated noise. Marine Ecology Progress Series, 538, 185–195. https://doi.org/10.3354/meps11468. |
⇡3 | Roberts, L., Cheesman, S., Elliott, M., & Breithaupt, T. (2016). Sensitivity of Pagurus bernhardus (L.) to substrate-borne vibration and anthropogenic noise. Journal of Experimental Marine Biology and Ecology, 474, 185–194. https://doi.org/10.1016/j.jembe.2015.09.014. |
⇡4 | Budelmann, B. U. (1992). Hearing in Nonarthropod Invertebrates. In D. B. Webster, A. N. Popper, & R. R. Fay (Eds.), The Evolutionary Biology of Hearing (pp. 141–155). Springer New York. https://doi.org/10.1007/978-1-4612-2784-7_10. |
⇡5 | Breithaupt, Th., & Tautz, J. (1988). Vibration sensitivity of the crayfish statocyst. Naturwissenschaften, 75(6), 310–312. https://doi.org/10.1007/BF00367325. |
⇡6 | Wiese, K. (1976). Mechanoreceptors for near-field water displacements in crayfish. Journal of Neurophysiology, 39(4), 816–833. https://doi.org/10.1152/jn.1976.39.4.816. |
⇡7 | Tautz, J., & Sandeman, D. C. (1980). The detection of waterborne vibration by sensory hairs on the chelae of the crayfish. Journal of Experimental Biology, 88(1), 351–356. https://doi.org/10.1242/jeb.88.1.351. |
⇡8, ⇡18 | Jézéquel, Y., Jones, I. T., Bonnel, J., Chauvaud, L., Atema, J., & Mooney, T. A. (2021). Sound detection by the American lobster ( Homarus americanus ). Journal of Experimental Biology, 224(6), jeb240747. https://doi.org/10.1242/jeb.240747. |
⇡9, ⇡19 | Budelmann, B. U. (1992). Hearing in Crustacea. In D. B. Webster, A. N. Popper, & R. R. Fay (Eds.), The Evolutionary Biology of Hearing (pp. 131–139). Springer New York. https://doi.org/10.1007/978-1-4612-2784-7_9. |
⇡10 | Cohen, M. J. (1955). The function of receptors in the statocyst of the lobster Homarus americanus. The Journal of Physiology, 130(1), 9–34. https://doi.org/10.1113/jphysiol.1955.sp005389. |
⇡11, ⇡15 | Cohen, M. J., & Dijkgraaf, S. (1961). Mechanoreception. In The Physiology of Crustacea (2nd ed., pp. 65–108). Academic Press. |
⇡12 | Lovell, J. M., Moate, R. M., Christiansen, L., & Findlay, M. M. (2006). The relationship between body size and evoked potentials from the statocysts of the prawn Palaemon serratus. Journal of Experimental Biology, 209(13), 2480–2485. https://doi.org/10.1242/jeb.02211. |
⇡13 | Mooney, T. A., Hanlon, R. T., Christensen-Dalsgaard, J., Madsen, P. T., Ketten, D. R., & Nachtigall, P. E. (2010). Sound detection by the longfin squid (Loligo pealeii) studied with auditory evoked potentials: Sensitivity to low-frequency particle motion and not pressure. Journal of Experimental Biology, 213(21), 3748–3759. https://doi.org/10.1242/jeb.048348. |
⇡14 | Hu, M. Y., Yan, H. Y., Chung, W.-S., Shiao, J.-C., & Hwang, P.-P. (2009). Acoustically evoked potentials in two cephalopods inferred using the auditory brainstem response (ABR) approach. Comparative Biochemistry and Physiology Part A: Molecular & Integrative Physiology, 153(3), 278–283. https://doi.org/10.1016/j.cbpa.2009.02.040. |
⇡16 | Lovell, J. M., Findlay, M. M., Moate, R. M., & Yan, H. Y. (2005). The hearing abilities of the prawn Palaemon serratus. Comparative Biochemistry and Physiology Part A: Molecular & Integrative Physiology, 140(1), 89–100. https://doi.org/10.1016/j.cbpb.2004.11.003. |
⇡17 | Dinh, J. P., & Radford, C. (2021). Acoustic particle motion detection in the snapping shrimp (Alpheus richardsoni). Journal of Comparative Physiology A, 207(5), 641–655. https://doi.org/10.1007/s00359-021-01503-4. |
⇡20 | Lacourse, J. R., & Northrop, R. B. (1978). A preliminary study of mechanoreceptors within the anterior byssus retractor muscle of Mytilus edulis L. The Biological Bulletin, 155(1), 161–168. https://doi.org/10.2307/1540873. |
⇡21 | Charifi, M., Sow, M., Ciret, P., Benomar, S., & Massabuau, J.-C. (2017). The sense of hearing in the Pacific oyster, Magallana gigas. PLOS ONE, 12(10), e0185353. https://doi.org/10.1371/journal.pone.0185353. |
⇡23, ⇡25, ⇡27, ⇡30 | Horch, K. (1971). An organ for hearing and vibration sense in the ghost crab Ocypode. Zeitschrift Fur Vergleichende Physiologie, 73(1), 1–21. https://doi.org/10.1007/BF00297698. |
⇡24, ⇡26, ⇡28 | Salmon, M., Horch, K., & Hyatt, G. W. (1977). Barth’s myochordotonal organ as a receptor for auditory and vibrational stimuli in fiddler crabs (Uca pugilator and U. minax). Marine Behaviour and Physiology, 4(3), 187–194. https://doi.org/10.1080/10236247709386951. |