How does sound propagate from air into water?
In the simplest situations, sound travels through a medium in straight lines. However, at some point, the medium may have changes in temperature, salinity, or pressure that cause the speed of sound to change. This change in sound speed will bend or refract the sound. Sound will bend towards the region with a slower sound speed.
In other instances, the sound may encounter a medium with a different density. A particularly important case is when airborne sound meets water. A portion of the sound wave will reflect away from the water and into the air, while another part will transmit into the water. During reflection the direction of the incoming wave changes. The original wave moving towards the boundary is called the incident wave. It hits the boundary at an angle, and its path of movement is redirected. The resulting reflected wave travels away from the boundary at an angle. A line perpendicular to the boundary, called a normal line, defines two angles: the angle of incidence, depicted in this figure as “∠I“, and the angle of reflection, depicted in this figure as “∠R“. The two angles are equal. A third angle often used in acoustics is the grazing angle, depicted in this figure as “∠G”, which is the complementary angle to the angle of incidence.
The sound wave can also be transmitted into the water. The amount of energy transmitted depends on the angle of incidence and the acoustic properties of the two media. More transmission relative to reflection occurs if the acoustic properties of the two media are similar. Because the sound speed in water is greater than the sound speed in air, a special angle of incidence called the critical angle exists. When the sound arrives at an incident angle that is greater than the critical angle, the sound is almost perfectly reflected (the blue line in the figure below). When the incident angle of the sound is less than the critical angle, a portion of the wave enters the water (the yellow line in the figure below). For the specific case of sound entering water from the air, the critical angle (θc) is about 15° relative to the normal line.
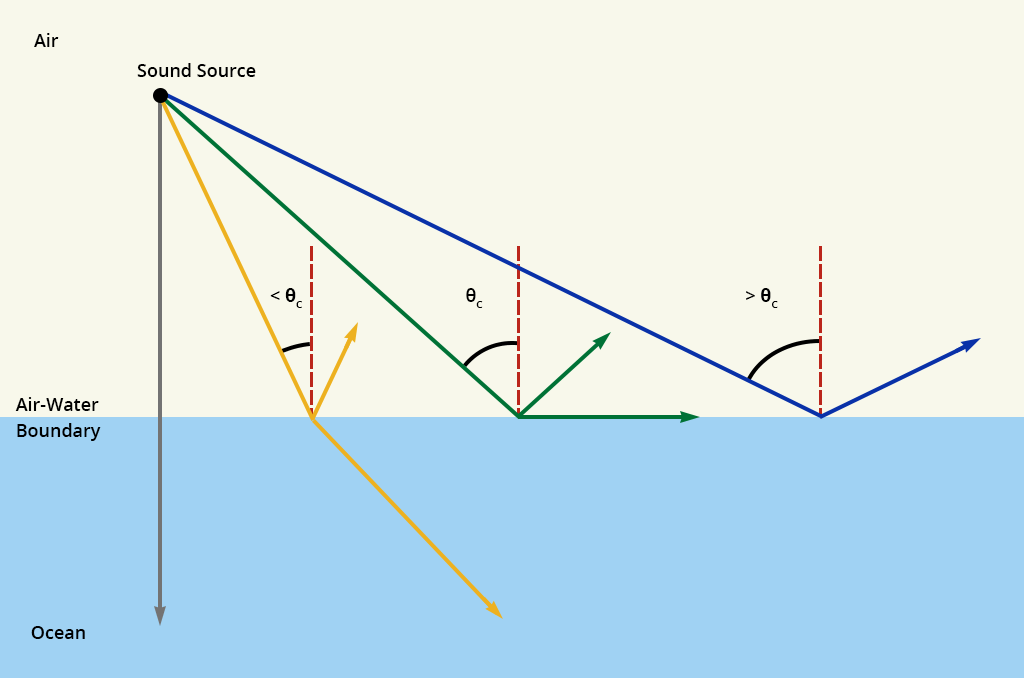
The resulting pathways at four different angles of incidence at the air-water boundary: at 90 degrees, less than the critical angle (< θc), at the critical angle (θc), and greater than the critical angle (> θc). Image credit URI.
This science becomes important when discussing how certain anthropogenic activities, such as wind energy production, may affect marine animals. There is concern about the acoustic pathways in which sound from wind turbines might enter the water and affect marine life. When the blades of a wind turbine are spinning, they produce low frequency sound that may enter the water through an air-to-water path. However, this transmission will only occur where sound energy reaches the air-water boundary at incident angles smaller than the critical angle. As a result, air-to-water transmission only takes place at distances close to the turbine.
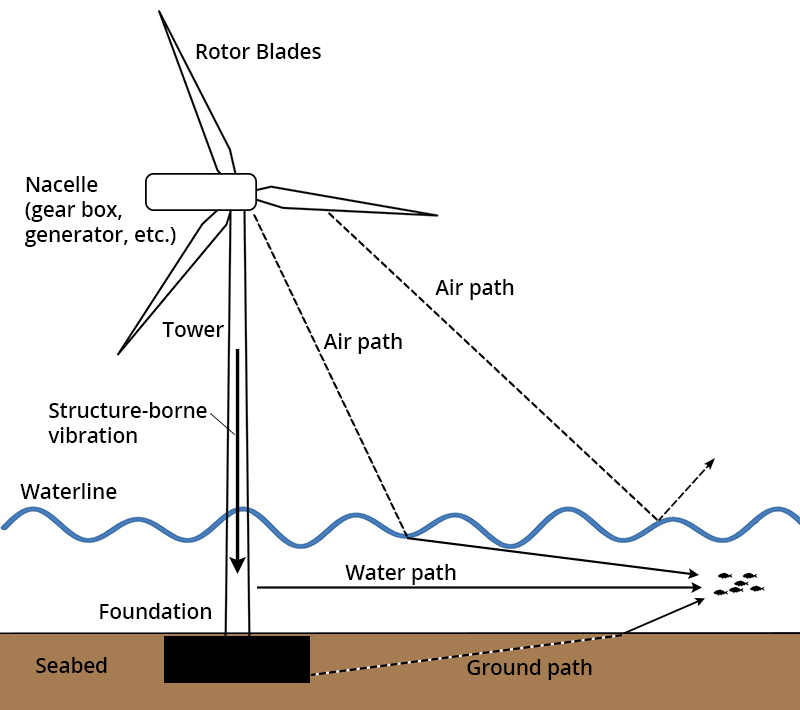
Acoustic pathways for underwater noise from an offshore wind turbine under operation. Kikuchi, R. (2010). Risk formulation for the sonic effects of offshore wind farms on fish in the EU region. Marine Pollution Bulletin, 60(2), 172–177.
Air-to-water transmission of sound is also an important issue when understanding underwater sound transmission from manned aircraft (including sonic booms), rocket launches, explosions, and even autonomous aerial vehicles (drones).
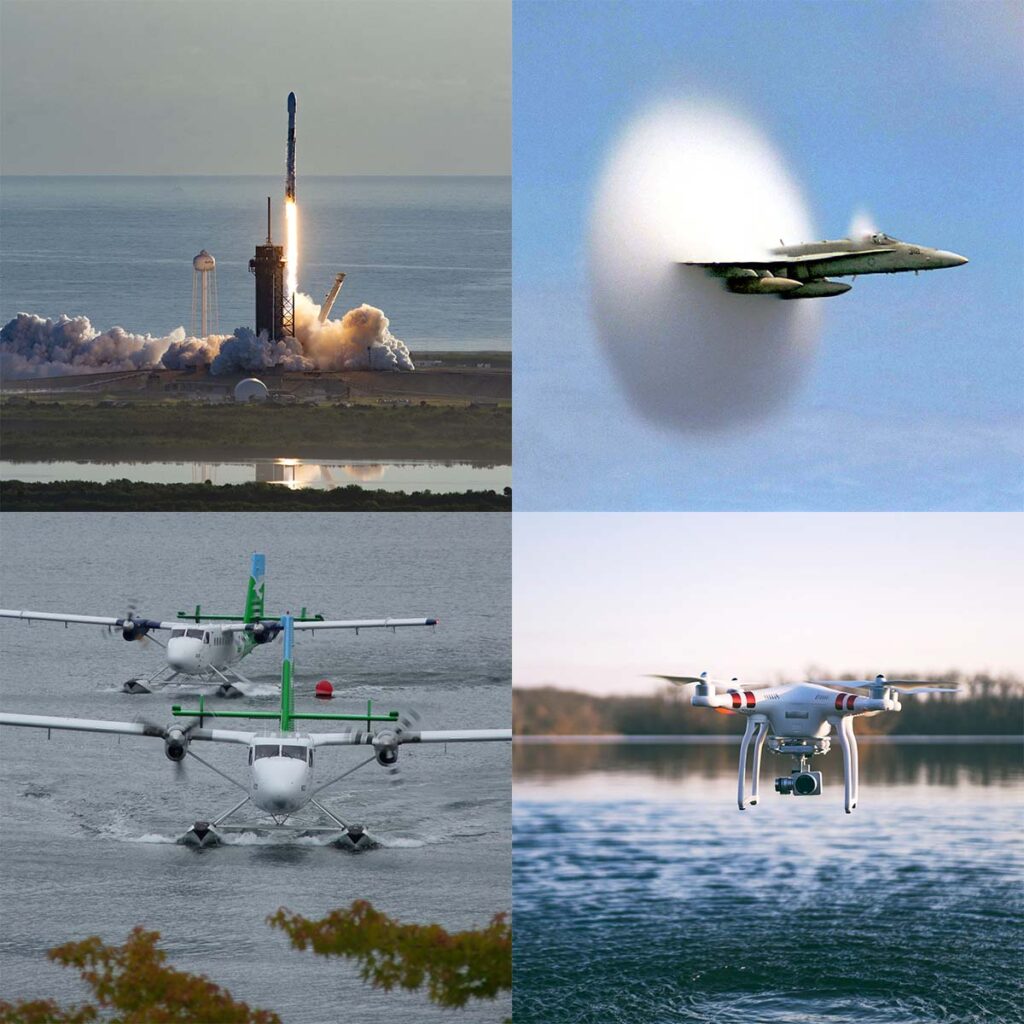
Top left: SpaceX launch, March 18, 2020. The Falcon 9 lifted off from Launch Complex 39A (LC-39A) at NASA’s Kennedy Space Center in Florida. Photo credit: Space X, CC BY-NC 2.0. Top right: A US Navy F/A-18 Hornet was photographed just as it broke the sound barrier. Photo Credit: Ensign John Gay, USS Constellation, US Navy, public domain. Bottom left: Sea Planes in Vancouver. Image Credit: Wikimedia Commons user FreebirdBiker, CC BY 3.0. Bottom right: Drone flying over water. Photo Credit: Aaron Burden, public domain.
Additional Links on DOSITS
- Science > Sound Movement > How fast does sound travel?
- Science > How does sound move? > Refraction
- Science > How does sound move? > Reflection
- Science > How does sound in air differ from sound in water?
- Animals > Anthropogenic Sound Sources > Wind Turbines
- Audio Gallery > Wind Turbine Sounds
References
- Chapman, D. M. F., & Ward, P. D. (1990). The normal‐mode theory of air‐to‐water sound transmission in the ocean. The Journal of the Acoustical Society of America, 87(2), 601–618. https://doi.org/10.1121/1.398929
- Chapman, D. M. F., Thomson, D. J., & Ellis, D. D. (1992). Modeling air‐to‐water sound transmission using standard numerical codes of underwater acoustics. The Journal of the Acoustical Society of America, 91(4), 1904–1910. https://doi.org/10.1121/1.403701
- Medwin, H., & Clay, C. S. (1998). Fundamentals of acoustical oceanography. Academic Press.
- Rossing, T. D. (Ed.). (2007). Springer handbook of acoustics. Springer.
- Sparrow, V. W. (1993). Sonic boom wave propagation from air into water: Implications for marine mammals. The Journal of the Acoustical Society of America, 94(3), 1850–1851. https://doi.org/10.1121/1.407693
- Urick, R. J. (1972). Noise Signature of an Aircraft in Level Flight over a Hydrophone in the Sea. The Journal of the Acoustical Society of America, 52(3B), 993–999. https://doi.org/10.1121/1.1913206
- Urick, R. J. (1983). Principles of Underwater Sound, Third Edition (3rd edition, Reprint 2013). McGraw-Hill, Inc.