Using acoustics to explore the unknown ocean
Aristotle (384–322 BC) was among the first to note that sound could be heard in water as well as in air. Nearly 2,000 years later, Leonardo da Vinci (1452-1519) made an observation that ships could be heard at great distances underwater (Bjørnø and Buckingham, 2017). In 1743, Abbé J. A. Nollet conducted a series of experiments to determine if sounds could travel through water. With his head underwater, he reported hearing a pistol shot, bell, whistle, and shouts. He also noted that an alarm clock clanging in water could be heard easily by an underwater observer, but not in air, clearly demonstrating sound travels through water. The first successful measurements of the speed of sound in water were not made until the early 1800s. Using a long tube to listen underwater, as suggested by da Vinci, Jean-Daniel Colladon and Charles-Franciois Stern in 1826 recorded how fast the sound of a submerged bell traveled across Lake Geneva. These early discoveries paved the way for the use of sound to explore under the sea.
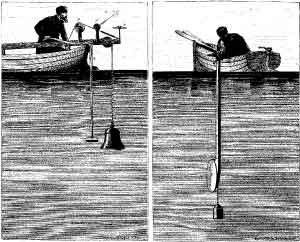
In 1826 on Lake Geneva, Switzerland, Jean-Daniel Colladon, a physicist, and Charles-Francois Sturm, a mathematician, made the first recorded attempt to determine the speed of sound in water. In their experiment, the underwater bell was struck simultaneously with ignition of gunpowder on the first boat. The sound of the bell and flash from the gunpowder were observed 10 miles away on the second boat. The time between the gunpowder flash and the sound reaching the second boat was used to calculate the speed of sound in water. Colladon and Sturm were able to determine the speed of sound in water fairly accurately with this method. J. D. Colladon, Souvenirs et Memoires, Albert-Schuchardt, Geneva, 1893.
One of the first applications of underwater sound to explore the unknown ocean was to determine the depth of the sea by listening for echoes. Previously, water depth was measured by lowering a weighted line from the deck of a ship, which is tedious, dangerous, and not very accurate. In 1838, Charles Bonnycastle performed the first known echosounding experiments. In 1859, Lt. Matthew Fontaine Maury, commander of the U.S. Navy Depot of Charts and Instruments, attempted, unsuccessfully, to use sound to measure the depth of the ocean. These experiments failed not because the idea was wrong, but because Lt. Maury did not have a means to detect the echo. Eventually, echosounding became one of the most important applications of underwater sound to explore the ocean.
Toward the end of the 1800s, increasing ship traffic raised concerns about navigation. Additionally,
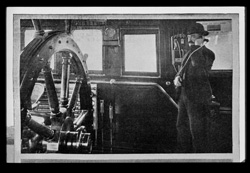
Demonstrating listening device, very similar to a telephone, used on board ships with submarine signaling apparatus. In: “Submarine Signaling,” Scientific American Supplement, No. 2071, pp. 168-170, Sept. 11, 1915. Image courtesy of NOAA Photo Library.
the bright lights and loud sirens emitted from lighthouses and lightships did not travel far enough to warn ships about the dangers of shallow waters and rocks. In 1889, the American Lighthouse Board mentioned an alternative underwater bell and microphone system devised by Lucien Blake. In 1901, a group of scientists, who believed that underwater sound would provide the most reliable warning, formed the Submarine Signal Company. In developing equipment to be used for increased navigational safety, the Company used underwater bells located under lightships or near lighthouses that could be detected by receivers installed on ships. The carbon-granule microphone developed by Thomas Edison and his collaborators for the first telephones was installed in a waterproof container, serving as the hydrophone to receive the underwater bell signals. Unfortunately, the ship-mounted hydrophones also picked up background noise, including ship machinery, splashing water, and fish, which made it sometimes difficult to hear the bells’ sounds.
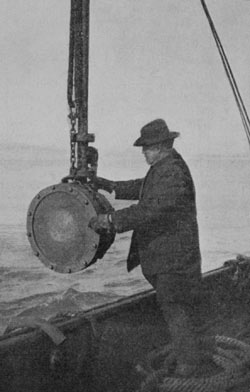
Reginald Fessenden and the Fessenden Oscillator. In “Submarine Signaling,” Scientific American Supplement, No. 2071, pp. 168-170, Sept. 11, 1915. Image courtesy of NOAA Photo Library.
The sinking of the passenger ship Titanic on April 14, 1912, emphasized how little was known about oceanic conditions. Within a week of the ship’s tragic collision with an iceberg, L. R. Richardson filed a patent for an invention that used sound and its echoes off objects to determine distances in air. This technique is called echo-ranging. A month later, he filed a patent application for doing the same thing underwater. However, at this time, a source more reliable than bells still did not exist. To meet the Submarine Signal Company’s desire for an improved hydrophone, Reginald Aubrey Fessenden designed an echo-ranging device. Resembling a high-powered underwater loudspeaker, it both produced and detected sounds and was later called the “Fessenden Oscillator.”
In January 1913, Richardson transmitted messages several miles between two tugboats in Boston Harbor using his new oscillator. A year later, Fessenden conducted echo-ranging trials. He was able to detect a 130-foot-high, 450-foot-long iceberg more than two miles away. He also successfully detected the seafloor at a depth of 31 fathoms (186 feet). Despite these encouraging results, the Submarine Signal Company decided not to market an echo-ranging system or an echosounder. It was not until 1923, following World War I (WWI), that the company put into production a low-frequency echosounder based on the Fessenden Oscillator. They called the echosounder a “fathometer” because depth was measured in fathoms. By the mid-1930s, practically every submarine used an underwater telegraph system based upon the Fessenden Oscillator.
The use of acoustics to explore the ocean increased after World War I. During the war, passive sonar systems were developed to detect German submarines. Researchers then determined if the transmitter and receiver (transducer) in a single-beam active sonar system were oriented toward the seafloor, water depth could be better determined. Also in this period, marine geologists determined that sonar systems could be used to detect layers of rocks and sediment below the ocean bottom. With multiple passes of sonar over an area, a profile could be constructed. This early work formed the foundation for being able to map the ocean floor, which continues today along with new technological developments.
Where Acoustic positioning (“underwater GPS”) and communication services have been installed across the globe, ocean basin-scale undersea navigation is possible. This aids management of floats, ocean gliders, and autonomous vehicles (AUVs) and is especially valuable in ice-covered polar regions (Graupe et al., 2022).
Developments in passive sonar systems include an individual hydrophone or an array of hydrophones, either towed behind a vessel, mounted to a ship’s hull, or fixed to a platform. Hydrophone arrays have continually evolved to improve the granularity of acoustic analyses. Passive Acoustic Monitoring (PAM) networks continue to monitor for and locate submarines and are used for a variety of applications.
Regional ambient sound analyses can provide a great deal of information about marine environments, including geophysical processes, such as earthquakes, volcanic activity, deep sea vents, ice formation, ice movement, and iceberg calving. Also, temperature differences can be detected through acoustic experiments, providing information about global climate change.
With different applications, various sonar systems are employed for a variety of needs and goals. Multibeam, side-scan, single-beam (split-beam), sub-bottom profiling, and synthetic aperture sonar are all examples of active sonar systems. For example, if the goal is to image a shipwreck or artifact, the proper tool would be a sonar system that is better at gathering data from a small area in very high resolution. If the goal is to produce maps of the seafloor and geological features, multibeam sonar systems are the appropriate tool.
Producing maps of the seafloor remains a high priority. In 2017 many partners across the world came together through the Nippon Foundation-GEBCO Seabed 2030 Project (Seabed 2030) effort. The goal of this project is to achieve a complete map of the entire seabed by 2030. When the effort started, only about 6% of the ocean floor had been mapped. As of 2024, over 25% of the seabed had been mapped, with resolutions as high as 100 meters. There are many international partners in this effort, as ensuring a fully mapped ocean will require cross sectoral, interdisciplinary collaboration, and partnerships worldwide.
An area of the ocean which is exceptionally dynamic and notoriously difficult to map with traditional sonar systems are the nearshore ocean environments or coastlines. As this area is the epicenter of human-ocean interaction, it is critical to obtain accurate information for coastal hazard assessment and mitigation, marine resource management, coastal engineering, and navigation safety. Light detection and ranging (lidar), is a remote sensing method that uses light in the form of a pulsed laser to measure ranges (variable distances) to Earth. A solution was to employ Airborne Lidar Bathymetry (ALB) which was developed in the 1970s to assist in the detection of underwater mines in a faster, safer and more efficient way. The technology has grown in utility to map the depth of nearshore ocean environments, however there are limitations to the depths that can be mapped. More recently, a method of coupling airborne lidar and vessel-based mapping is rapidly becoming a very effective means for fast and high-resolution coastal mapping.
Resources
- Bjørnø, L., Buckingham, M.J. Chapter 1 – General Characteristics of the Underwater Environment, Editor(s): Thomas H. Neighbors, David Bradley, Applied Underwater Acoustics, Elsevier, 2017, Pages 1-84,ISBN 9780128112403, https://doi.org/10.1016/B978-0-12-811240-3.00001-1.
- Cristian Graupe, Lora J. Van Uffelen, Peter F. Worcester, Matthew A. Dzieciuch, Bruce M. Howe; An automated framework for long-range acoustic positioning of autonomous underwater vehicles. J. Acoust. Soc. Am. 1 September 2022; 152 (3): 1615–1626. https://doi.org/10.1121/10.0013830
- Howe B.M., Miksis-Olds J., Rehm E., Sagen H., Worcester P.F. and Haralabus G. (2019) Observing the Oceans Acoustically. Front. Mar. Sci. 6:426. https://doi.org/10.3389/fmars.2019.00426
- Miksis-Olds J., Howe B.M., Tyack, P. (2018) Exploring the Ocean Through Soundscapes. Acoustics Today. Vol. 14, Issue 1. http://acousticstoday.org/exploring-ocean-soundscapes/
- Nippon Foundation – GEBCO Seabed 2030 (https://seabed2030.org/)
- Shell Ocean Discovery XPRIZE (https://www.xprize.org/prizes/ocean-discovery)