Pile driving
Constructing large, marine structures, such as bridges, wharfs, wind turbines, and offshore oil and gas structures requires supports, called piles, that are driven into the seafloor. These piles, most often made of steel, though they can be made of wood or reinforced concrete, can penetrate the bottom for tens of meters, often until they hit bedrock. One common method to drive piles is impact pile driving where a hydraulic hammer repeatedly strikes the top of the pile, approximately once per second. Depending on the diameter of the pile, size of the hammer, bottom properties, and the required penetration depth, it may take several hours and as many as 5,000 strikes to drive one pile into the seafloor.
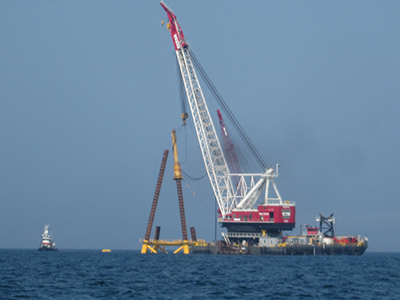
Pile driving activity associated with wind farm construction off the coast of Block Island, Rhode Island. The hydraulic hammer is the yellow structure at the top of the pile. Most piles are driven vertically but this construction used piles driven at an angle. Photo courtesy of Dr. Kathleen Vigness-Raposa.
Sound is directly radiated from the pile into the surrounding water, as well as through the seafloor. The figure that follows shows a pile being driven. The arrow indicates a strike by a hammer on the pile (thick, black, vertical bar), which causes the pile to bulge and produce a sound wave in water, a seismic wave in the sediment, and an interface wave where the water and seafloor meet. Sounds propagated through the seafloor may return to the water column, perhaps at considerable distances from the pile being driven.
The specific characteristics of the sounds generated depend on the pile and hammer characteristics. The propagation of these sounds depends on several factors, including bottom type, seafloor penetration depth, water depth, and oceanographic conditions.
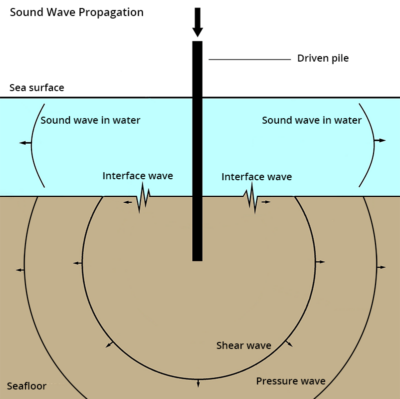
Diagram of sound waves generated by pile driving. Sound waves in water generated by pile driving can come directly from the pile in water, from seismic waves in the seafloor as the seismic waves reach the sediment water interface, and from interface waves that move away from the pile along the water-seafloor interface. Image modified from Dr. Anthony D. Hawkins.
Impact pile driving produces intense, broadband impulsive sounds that can propagate many kilometers from the impact location. At close ranges, these sounds are characterized by a short rise time to a maximum pressure, followed by a rapid decrease to a minimum pressure, which then continues as a diminishing, oscillating pressure. The frequency characteristics of the pile driving signals change with increasing range from the impact location. Close to the pile, the signals are relatively broadband (from below 10 Hz to over 3 kHz), as can be seen in the spectrogram that follows. Further away the signals are dominated by low frequency components (less than 1 kHz). However, water depth affects how the sound propagates; at longer ranges, there is a cut-off frequency below which sound is radiated into the seafloor and does not propagate horizontally. For example, in the construction of the Block Island Wind Farm, assuming a water sound speed of 1500 m/s, a bottom sound speed of 1700 m/s, and a water depth of 27 m, the cutoff frequency is about 30 Hz.
There are three common metrics for characterizing pile driving sounds: peak pressure, single strike sound exposure level (SELss), and root-mean-square pressure (sound pressure level, SPL). For more information, please see the Advanced Topic on Sound Pressure Levels and Sound Exposure Levels. The peak pressure, also called the 0-to-peak pressure, is the range in pressure between zero and the greatest pressure of the signal. The root-mean-square pressure (abbreviated as rms pressure) is the square root of the average of the square of the pressure of the sound signal over a given duration. Because there is no standard duration for measuring rms pressure, rms pressure will vary dramatically depending on the duration over which the signal is averaged and is not considered an appropriate metric for impulsive sounds.
Peak pressure and SELss are most appropriate for describing the impulsive sounds from pile driving. Peak pressure is important because a rapid change in pressure can potentially affect marine animals. At close ranges (e.g., within tens of meters of the impact location) sound pressure levels (SPLs) range up to 220 dB peak re 1 uPa, but the exact value may vary substantially depending on the size and power of the hammer, pile material and diameter, and seafloor properties.
Piles can also be “shaken” into the sediment by vibratory pile driving, which is not an impulsive sound source. This produces a continuous sound with peak pressures lower than those generated by impact pile driving. Studies are just beginning to be conducted to determine if and how vibratory pile driving might affect marine animals[1]Branstetter, B. K., Bowman, V. F., Houser, D. S., Tormey, M., Banks, P., Finneran, J. J., & Jenkins, K. (2018). Effects of vibratory pile driver noise on echolocation and vigilance in bottlenose dolphins (Tursiops truncatus). The Journal of the Acoustical Society of America, 143(1), 429–439. https://doi.org/10.1121/1.5021555.. While it may seem logical to replace impact hammering with vibratory pile driving, vibratory pile driving cannot drive piles nearly as deep or in as dense a substrate as hammering.
Two criteria for assessing the potential impacts of pile driving sounds are: 1) SPL peak and 2) single strike sound exposure level (SELss). In some cases, the accumulated energy from multiple strikes, the SELcum, may be used in evaluating potential impacts. Again, for more information, please see the Advanced Topic on Sound Pressure Levels and Sound Exposure Levels.
Potential Effects:Fishes
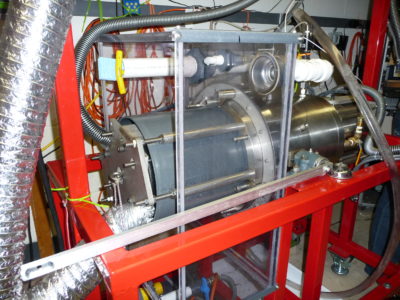
High Intensity Controlled Impedance Fluid-filled wave Tube (HICI-FT) chamber used to investigate the potential effects of pile driving on fishes. The system enabled the simulation of pile driving sounds in a laboratory setting and also provided control of the parameters that affect pile driving signals. Copyright 2019 Arthur Popper, all rights reserved.
To investigate the potential effects of pile driving on fishes, several species of freshwater fish including Chinook salmon, were exposed in a chamber to simulated pile driving sounds. The scientists reported that injuries varied according to the peak pressure, number of strikes, the species of fish, and whether a species had a swim bladder. The scientists found that at the lowest sound pressure levels and fewest number of pile strikes there were no detectable injuries[2]Casper, B. M., Halvorsen, M. B., Carlson, T. J., & Popper, A. N. (2017). Onset of barotrauma injuries related to number of pile driving strike exposures in hybrid striped bass. The Journal of the Acoustical Society of America, 141(6), 4380–4387. https://doi.org/10.1121/1.4984976.. Tissue damage increased both as energy accumulated over multiple strikes (SELcum) and/or as single strike energy increased. Injuries reported included damage to hair cells, swim bladders, and soft tissues, according to the exposure level [3]Halvorsen, M. B., Casper, B. M., Woodley, C. M., Carlson, T. J., & Popper, A. N. (2012). Threshold for Onset of Injury in Chinook Salmon from Exposure to Impulsive Pile Driving Sounds. PLoS ONE, 7(6), e38968. https://doi.org/10.1371/journal.pone.0038968.[4]Casper, B. M., Smith, M. E., Halvorsen, M. B., Sun, H., Carlson, T. J., & Popper, A. N. (2013). Effects of exposure to pile driving sounds on fish inner ear tissues. Comparative Biochemistry and Physiology Part A: Molecular & Integrative Physiology, 166(2), 352–360. https://doi.org/10.1016/j.cbpa.2013.07.008.. These studies exposed fishes to 960 or 1920 pile strikes, a “worst-case scenario” assuming fishes remain in an area of pile driving, something that may not occur in the wild. Additional data are needed to understand the potential effects on fishes when exposed to fewer pile strikes and to estimate potential impact if fishes depart the area of pile driving. Data are also needed to determine the effects of particle motion produced by pile driving on fishes.
Hybrid striped bass were exposed in the same chamber to simulated pile driving sounds. With sound exposure level for a single strike (SELss) of 183 dB, mortal injuries (ruptured swim bladder and/or kidney hemorrhage) were observed in fishes exposed to as few as 96 pile strikes[5]Casper, B. M., Halvorsen, M. B., Carlson, T. J., & Popper, A. N. (2017). Onset of barotrauma injuries related to number of pile driving strike exposures in hybrid striped bass. The Journal of the Acoustical Society of America, 141(6), 4380–4387. https://doi.org/10.1121/1.4984976.. Physical injuries sustained by the fish increased in number and severity as the energy in each pile strike (SELss) and the number of pile strikes increased, calculated as cumulative sound exposure level (SELcum). The lowest injury probabilities were associated with the lowest SELss and lowest number of strikes, and the highest injury probability with the highest SELss and highest number of strikes.
Data on fishes’ behavioral reactions to pile driving sounds is limited. Behavioral effects were seen in schools of free-swimming sprat (related to herring) and Atlantic mackerel (distantly related to tuna) exposed to short sequences of repeated impulsive sounds that simulated the strikes from an impact pile driver. Behavioral responses included both the break up of fish schools and changes in the depth of the schools. The incidence of responses increased with increasing sound level, with sprat schools being more likely to disperse and mackerel schools more likely to change depth[6]Hawkins, A. D., Roberts, L., & Cheesman, S. (2014). Responses of free-living coastal pelagic fish to impulsive sounds. The Journal of the Acoustical Society of America, 135(5), 3101–3116. https://doi.org/10.1121/1.4870697..
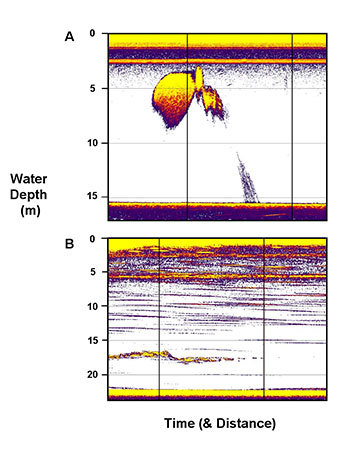
Responses of a sprat school (A, at 5 m depth) and a mackerel school (B, at 17 m depth) to sound playback, observed on an echo sounder. The sound was a 20 s sequence of 10 low frequency impulses, simulating the sound propagated several kilometres away from a pile driver or seismic air gun, occurring between the two vertical lines. After a short latency the sprat school breaks up and the fish disperse down to the seabed. The mackerel school changes depth before dispersing laterally.Reprinted with permission from Hawkins AD, Popper AN (2014) Assessing the impacts of underwater sounds on fishes and other forms of marine life. Acoustics Today 10(2):30–41.
Many behavioral studies have been conducted in a laboratory setting and these studies should be considered with care since fish in a tank, just as wild animals confined to cages, may not behave the same as they would in the wild. Thus, research is needed on free-swimming animals to determine the potential for behavioral effects. Furthermore, no data are available on temporary threshold shift (TTS) or masking for fish exposed to pile driving. Responses to a signal may vary within a species, and even a single animal, depending on things such as hearing sensitivity, sex, age, size, and motivation (feeding, mating, transiting, etc.)
Particle motion most likely also plays a role in fishes’ response to pile driving, especially close to the source (in the near field), though data are limited. Animals near the seabed may detect not only water-borne sounds, but also sound that propagates through the substrate and re-enters the water.
Potential Effects: Marine Mammals
Research has been conducted and guidelines developed for pile driving for many marine areas. Research results have shown that pile driving sounds may cause temporary threshold shift (TTS)[7]Kastelein, R. A., Gransier, R., Marijt, M. A. T., & Hoek, L. (2015). Hearing frequency thresholds of harbor porpoises (Phocoena phocoena) temporarily affected by played back offshore pile driving sounds. The Journal of the Acoustical Society of America, 137(2), 556–564. https://doi.org/10.1121/1.4906261., and by extrapolation, permanent threshold shift (PTS)[8]Hastie, G. D., Russell, D. J. F., McConnell, B., Moss, S., Thompson, D., & Janik, V. M. (2015). Sound exposure in harbour seals during the installation of an offshore wind farm: predictions of auditory damage. Journal of Applied Ecology, 52(3), 631–640. https://doi.org/10.1111/1365-2664.12403.. TTS was measured in two captive harbor porpoises after being exposed to recorded pile-driving sounds[9]Kastelein, R. A., Helder-Hoek, L., Covi, J., & Gransier, R. (2016). Pile driving playback sounds and temporary threshold shift in harbor porpoises ( Phocoena phocoena ): Effect of exposure duration. The Journal of the Acoustical Society of America, 139(5), 2842–2851. https://doi.org/10.1121/1.4948571.. The average received single-strike sound exposure levels (SELss) was 145 dB re 1 µPa2s, with exposure durations of 15 minutes to 6 hours (SELcum ranged from 173 to 187 dB re 1 µPa2s). Mean TTS increased from 0 dB after 15 minutes of exposure to 5 dB after 6 hours of exposure.
Scientists measured pile driving sounds during construction of a wind farm off the northeast coast of Scotland. Based on regulatory criteria[10]Bailey, H., Senior, B., Simmons, D., Rusin, J., Picken, G., & Thompson, P. M. (2010). Assessing underwater noise levels during pile-driving at an offshore windfarm and its potential effects on marine mammals. Marine Pollution Bulletin, 60(6), 888–897. https://doi.org/10.1016/j.marpolbul.2010.01.003., the peak broadband sound levels expected to result in TTS onset (224 dB re 1 µPa and 212 dB re 1 µPa) would have been exceeded within 10 m of the pile driving operations for cetaceans and within 40 m for pinnipeds, respectively. The peak broadband levels estimated to cause PTS onset (230 dB re 1 µPa and 218 dB re 1 µPa) would occur within 5 m for cetaceans and 20 m for pinnipeds, respectively. Scientists determined that no form of injury or hearing impairment would occur at ranges greater than 100 m.
Observed behavioral responses in some marine mammals in association with pile driving include changes in swimming direction and/or speed, dive profiles, group movements, vocalizations, and respiration rates[11]Teilmann, J., & Carstensen, J. (2012). Negative long term effects on harbour porpoises from a large scale offshore wind farm in the Baltic—evidence of slow recovery. Environmental Research Letters, 7(4), 045101. https://doi.org/10.1088/1748-9326/7/4/045101.[12]Russell et al. (2016). Avoidance of wind farms by harbor seals is limited to pile driving activities. Journal of Applied Ecology. DOI: 10.1111/1365-2664.12678.[13]Haelters, J., Dulière, V., Vigin, L., & Degraer, S. (2015). Towards a numerical model to simulate the observed displacement of harbour porpoises Phocoena phocoena due to pile driving in Belgian waters. Hydrobiologia, 756(1), 105–116. https://doi.org/10.1007/s10750-014-2138-4.[14]Kastelein, R. A., van Heerden, D., Gransier, R., & Hoek, L. (2013). Behavioral responses of a harbor porpoise (Phocoena phocoena) to playbacks of broadband pile driving sounds. Marine Environmental Research, 92, 206–214. https://doi.org/10.1016/j.marenvres.2013.09.020.[15]Carstensen, J., Henriksen, O., & Teilmann, J. (2006). Impacts of offshore wind farm construction on harbour porpoises: Acoustic monitoring of echolocation activity using porpoise detectors (T-PODs). Marine Ecology Progress Series, 321, 295–308. https://doi.org/10.3354/meps321295.[16]Tougaard, J., Carstensen, J., Teilmann, J., Skov, H., & Rasmussen, P. (2009). Pile driving zone of responsiveness extends beyond 20 km for harbor porpoises ( Phocoena phocoena (L.)). The Journal of the Acoustical Society of America, 126(1), 11–14. https://doi.org/10.1121/1.3132523.. There are concerns that avoidance responses to pile driving may cause displacement from key habitats and/or impact foraging, nursing, and/or mating activities, and therefore the overall fitness, of a marine mammal. Harbor porpoises have been observed to be displaced at distances of greater than 20 kilometers during wind farm installations[17]Tougaard, J., Carstensen, J., Teilmann, J., Skov, H., & Rasmussen, P. (2009). Pile driving zone of responsiveness extends beyond 20 km for harbor porpoises ( Phocoena phocoena (L.)). The Journal of the Acoustical Society of America, 126(1), 11–14. https://doi.org/10.1121/1.3132523.. Significant population consequences could occur as a result of these behavioral responses. Additional work is required, however, to fully understand how received sound levels influence displacement (in the short and long term) and how this may vary with location and behavioral context. Long-term assessments are also necessary in order to understand potential impacts at the population level.
Mitigation Measures
Various mitigation measures, such as bubble curtains, screens, cofferdams (an insulating sleeve around the pile) or combinations of these measures, have been developed to diminish the potential impacts of pile driving on marine life. Acoustic deterrent devices have also been deployed prior to pile driving activities at many European wind farm sites in order to deter marine mammals from entering the construction zone. Pile driving activities may also begin with a “ramp-up” or “soft start” to keep marine mammals away from the activity. In these cases, lower hammer energy levels are used to start the pile driving process, and then the force of pile driving is gradually increased. Breaks in pile driving activities, to allow animals to travel through and/or return to an area, may also be important (Russell et al. 2016). This is especially important in areas where multiple wind farms are due for development at the same time.
Listen to the sound below to hear pile driving activity before and after a bubble curtain system is activated. Please note, this sound recording demonstrates the effectiveness of this mitigation option and is not meant to demonstrate how it is actually done (e.g., the bubble curtain would already be up and operational before any piling started in a real-world scenario)
Sound recording of 36-inch diameter steel pipe (1” wall thickness) being driven by a diesel impact hammer at a water depth of 7.5m. Approximately midway through the recording, a dual-ring bubble curtain (400 cubic ft per minute flow rate, 75 psi air pressure) is activated. One should be able to hear a difference in the pile-driving sounds before and after the bubble curtain is activated. The sounds were recorded by a hydrophone in 20m of water, approximately 1m above the seabed. Courtesy of Connor, Heithaus, Berggren and Miksis.
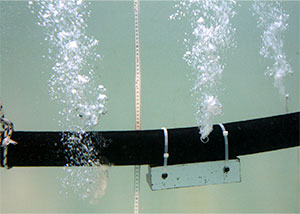
Close-up view of a bubble curtain in operation. One can see the streams of bubbles exiting the hose of the bubble curtain. Image courtesy of Georg Nehls.
Additional Links on DOSITS
- Advanced Topic > Sound pressure levels and sound exposure levels
- Advanced Topic > Propagation from a sound source array in the near field and far field
- Science > How does sound propagate through sediment?
- Animals and Sound > Behavioral changes in mammals
- Animals and Sound > Behavioral changes in fishes
- Animals and Sound > Masking
- Animals and Sound > Moderate or eliminate the effects of human activities
- People and Sound > How is sound used to protect marine mammals?
- People and Sound > How is sound used to research wind energy?
- Audio Gallery > Pile-driving
- Audio Gallery > Wind turbines
- Audio Gallery > Bubble curtain
- Webinar Archive > Pile Driving/Wind Turbines
Additional Resources
- Dahl, P. H., de Jong, C. A. F., & Popper, A. N. (2015). The Underwater Sound Field from Impact Pile Driving and Its Potential Effects on Marine Life. Acoustics Today, 11(2), 18–25. (PDF)
- De Jong, C. A. F., & Ainslie, M. A. (2008). Underwater radiated noise due to the piling for the Q7 Offshore Wind Park. The Journal of the Acoustical Society of America, 123(5), 2987–2987. https://doi.org/10.1121/1.2932518.
- Halvorsen, M. B., Casper, B. M., Woodley, C. M., Carlson, T. J., & Popper, A. N. (2011). Predicting and mitigating hydroacoustic impacts on fish from pile installations. NCHRP Research Results Digest 363, Project 25-28. Washington, D.C.: National Cooperative Highway Research Program, Transportation Research Board,National Academies Press. (Publication Link).
- Popper A.N. and Hawkins A.D. (2019). Impacts of Anthropogenic Noise on Fishes. Fisheries Society of the British Isles, Briefing Note. (Publication Link).
- BOEM Center for Marine Acoustics: BOEM Sound Source List March 2023 document (see BOEM Center for Marine Acoustics for additional information and questions about this document).
References
- Bailey, H., Brookes, K. L., & Thompson, P. M. (2014). Assessing environmental impacts of offshore wind farms: lessons learned and recommendations for the future. Aquatic Biosystems, 10(1), 8. https://doi.org/10.1186/2046-9063-10-8.
- Bailey, H., Senior, B., Simmons, D., Rusin, J., Picken, G., & Thompson, P. M. (2010). Assessing underwater noise levels during pile-driving at an offshore windfarm and its potential effects on marine mammals. Marine Pollution Bulletin, 60(6), 888–897. https://doi.org/10.1016/j.marpolbul.2010.01.003.
- Brandt, M., Diederichs, A., Betke, K., & Nehls, G. (2011). Responses of harbour porpoises to pile driving at the Horns Rev II offshore wind farm in the Danish North Sea. Marine Ecology Progress Series, 421, 205–216. https://doi.org/10.3354/meps08888.
- Casper, B. M., Halvorsen, M. B., Matthews, F., Carlson, T. J., & Popper, A. N. (2013). Recovery of barotrauma injuries resulting from exposure to pile driving sound in two sizes of hybrid striped bass. PLoS ONE, 8(9), e73844. https://doi.org/10.1371/journal.pone.0073844.
-
Dähne, M., Tougaard, J., Carstensen, J., Rose, A., & Nabe-Nielsen, J. (2017). Bubble curtains attenuate noise from offshore wind farm construction and reduce temporary habitat loss for harbour porpoises. Marine Ecology Progress Series, 580, 221–237. https://doi.org/10.3354/meps12257.
- Dolman, S., & Simmonds, M. (2010). Towards best environmental practice for cetacean conservation in developing Scotland’s marine renewable energy. Marine Policy, 34(5), 1021–1027. https://doi.org/10.1016/j.marpol.2010.02.009.
- Halvorsen, M. B., Casper, B. M., Matthews, F., Carlson, T. J., & Popper, A. N. (2012). Effects of exposure to pile-driving sounds on the lake sturgeon, Nile tilapia and hogchoker. Proceedings of the Royal Society B: Biological Sciences, 279(1748), 4705–4714. https://doi.org/10.1098/rspb.2012.1544.
-
Herbert-Read, J. E., Kremer, L., Bruintjes, R., Radford, A. N., & Ioannou, C. C. (2017). Anthropogenic noise pollution from pile-driving disrupts the structure and dynamics of fish shoals. Proceedings of the Royal Society B: Biological Sciences, 284(1863), 20171627. https://doi.org/10.1098/rspb.2017.1627.
- Kastelein, R. A., Schop, J., Gransier, R., & Hoek, L. (2014). Frequency of greatest temporary hearing threshold shift in harbor porpoises ( Phocoena phocoena ) depends on the noise level. The Journal of the Acoustical Society of America, 136(3), 1410–1418. https://doi.org/10.1121/1.4892794.
- Madsen, P., Wahlberg, M., Tougaard, J., Lucke, K., & Tyack, P. (2006). Wind turbine underwater noise and marine mammals: implications of current knowledge and data needs. Marine Ecology Progress Series, 309, 279–295. https://doi.org/10.3354/meps309279.
- Popper, A. N., Hawkins, A. D., Fay, R. R., Mann, D., Bartol, S., Carlson, T., Coombs, S., Ellison, W. T., Gentry, R., Halvorsen, M. B., Løkkeborg, S., Rogers, P., Southall, B. L., Zeddies, D., & Tavolga, W. N. (2014). Sound exposure guidelines for fishes and sea turtles: ASA S3/SC1.4 TR-2014 ; a technical report prepared by ANSI-accredited Standards Committee S3/SC1 and registered with ANSI. Springer.
- Popper, A. N., & Hawkins, A. D. (2018). The importance of particle motion to fishes and invertebrates. The Journal of the Acoustical Society of America, 143(1), 470–488. https://doi.org/10.1121/1.5021594.
- Reyff, J. (2012). Underwater Sounds From Unattenuated and Attenuated Marine Pile Driving. In A. N. Popper & A. Hawkins (Eds.), The Effects of Noise on Aquatic Life (Vol. 730, pp. 439–444). New York, NY: Springer New York. https://doi.org/10.1007/978-1-4419-7311-5_99.
- Ruppel, C. D., Weber, T. C., Staaterman, E. R., Labak, S. J., & Hart, P. E. (2022). Categorizing Active Marine Acoustic Sources Based on Their Potential to Affect Marine Animals. Journal of Marine Science and Engineering, 10(9), 1278. https://doi.org/10.3390/jmse10091278.
- Smith, M. E., Coffin, A. B., Miller, D. L., & Popper, A. N. (2006). Anatomical and functional recovery of the goldfish (Carassius auratus) ear following noise exposure. Journal of Experimental Biology, 209(21), 4193–4202. https://doi.org/10.1242/jeb.02490.
- Thompson, P. M., Lusseau, D., Barton, T., Simmons, D., Rusin, J., & Bailey, H. (2010). Assessing the responses of coastal cetaceans to the construction of offshore wind turbines. Marine Pollution Bulletin, 60(8), 1200–1208. https://doi.org/10.1016/j.marpolbul.2010.03.030.
Cited References
⇡1 | Branstetter, B. K., Bowman, V. F., Houser, D. S., Tormey, M., Banks, P., Finneran, J. J., & Jenkins, K. (2018). Effects of vibratory pile driver noise on echolocation and vigilance in bottlenose dolphins (Tursiops truncatus). The Journal of the Acoustical Society of America, 143(1), 429–439. https://doi.org/10.1121/1.5021555. |
---|---|
⇡2, ⇡5 | Casper, B. M., Halvorsen, M. B., Carlson, T. J., & Popper, A. N. (2017). Onset of barotrauma injuries related to number of pile driving strike exposures in hybrid striped bass. The Journal of the Acoustical Society of America, 141(6), 4380–4387. https://doi.org/10.1121/1.4984976. |
⇡3 | Halvorsen, M. B., Casper, B. M., Woodley, C. M., Carlson, T. J., & Popper, A. N. (2012). Threshold for Onset of Injury in Chinook Salmon from Exposure to Impulsive Pile Driving Sounds. PLoS ONE, 7(6), e38968. https://doi.org/10.1371/journal.pone.0038968. |
⇡4 | Casper, B. M., Smith, M. E., Halvorsen, M. B., Sun, H., Carlson, T. J., & Popper, A. N. (2013). Effects of exposure to pile driving sounds on fish inner ear tissues. Comparative Biochemistry and Physiology Part A: Molecular & Integrative Physiology, 166(2), 352–360. https://doi.org/10.1016/j.cbpa.2013.07.008. |
⇡6 | Hawkins, A. D., Roberts, L., & Cheesman, S. (2014). Responses of free-living coastal pelagic fish to impulsive sounds. The Journal of the Acoustical Society of America, 135(5), 3101–3116. https://doi.org/10.1121/1.4870697. |
⇡7 | Kastelein, R. A., Gransier, R., Marijt, M. A. T., & Hoek, L. (2015). Hearing frequency thresholds of harbor porpoises (Phocoena phocoena) temporarily affected by played back offshore pile driving sounds. The Journal of the Acoustical Society of America, 137(2), 556–564. https://doi.org/10.1121/1.4906261. |
⇡8 | Hastie, G. D., Russell, D. J. F., McConnell, B., Moss, S., Thompson, D., & Janik, V. M. (2015). Sound exposure in harbour seals during the installation of an offshore wind farm: predictions of auditory damage. Journal of Applied Ecology, 52(3), 631–640. https://doi.org/10.1111/1365-2664.12403. |
⇡9 | Kastelein, R. A., Helder-Hoek, L., Covi, J., & Gransier, R. (2016). Pile driving playback sounds and temporary threshold shift in harbor porpoises ( Phocoena phocoena ): Effect of exposure duration. The Journal of the Acoustical Society of America, 139(5), 2842–2851. https://doi.org/10.1121/1.4948571. |
⇡10 | Bailey, H., Senior, B., Simmons, D., Rusin, J., Picken, G., & Thompson, P. M. (2010). Assessing underwater noise levels during pile-driving at an offshore windfarm and its potential effects on marine mammals. Marine Pollution Bulletin, 60(6), 888–897. https://doi.org/10.1016/j.marpolbul.2010.01.003. |
⇡11 | Teilmann, J., & Carstensen, J. (2012). Negative long term effects on harbour porpoises from a large scale offshore wind farm in the Baltic—evidence of slow recovery. Environmental Research Letters, 7(4), 045101. https://doi.org/10.1088/1748-9326/7/4/045101. |
⇡12 | Russell et al. (2016). Avoidance of wind farms by harbor seals is limited to pile driving activities. Journal of Applied Ecology. DOI: 10.1111/1365-2664.12678. |
⇡13 | Haelters, J., Dulière, V., Vigin, L., & Degraer, S. (2015). Towards a numerical model to simulate the observed displacement of harbour porpoises Phocoena phocoena due to pile driving in Belgian waters. Hydrobiologia, 756(1), 105–116. https://doi.org/10.1007/s10750-014-2138-4. |
⇡14 | Kastelein, R. A., van Heerden, D., Gransier, R., & Hoek, L. (2013). Behavioral responses of a harbor porpoise (Phocoena phocoena) to playbacks of broadband pile driving sounds. Marine Environmental Research, 92, 206–214. https://doi.org/10.1016/j.marenvres.2013.09.020. |
⇡15 | Carstensen, J., Henriksen, O., & Teilmann, J. (2006). Impacts of offshore wind farm construction on harbour porpoises: Acoustic monitoring of echolocation activity using porpoise detectors (T-PODs). Marine Ecology Progress Series, 321, 295–308. https://doi.org/10.3354/meps321295. |
⇡16, ⇡17 | Tougaard, J., Carstensen, J., Teilmann, J., Skov, H., & Rasmussen, P. (2009). Pile driving zone of responsiveness extends beyond 20 km for harbor porpoises ( Phocoena phocoena (L.)). The Journal of the Acoustical Society of America, 126(1), 11–14. https://doi.org/10.1121/1.3132523. |