Distributed acoustic sensing (DAS): Shedding light on passive acoustics – September 20, 2023
A network of fiber-optic telecommunication cables spans 1.2 million km of the seafloor, covering both deep ocean and coastal areas around the globe. The cables are used to transmit phone calls, internet signals, and other data. Using a method called Distributed Acoustic Sensing (DAS), researchers are now working to repurpose these globally available telecommunication cables for real-time, underwater acoustics monitoring. Using DAS, acoustic signals can be measured over large distances (up to 200 kilometers), with high spatial resolution (up to a few meters), and a broad frequency band from <0.001 Hz to >1 kHz depending on system configuration[1]Lindsey, N. J., & Martin, E. R. (2021). Fiber-Optic Seismology. Annual Review of Earth and Planetary Sciences, 49(1), 309–336. https://doi.org/10.1146/annurev-earth-072420-065213..
Spare fibers are often installed within telecommunication cable bundles. DAS utilizes a device called an “interrogator” to connect to these unused cables and transforms their entire length into a continuous sensing element, all without disrupting the main data stream. The interrogator does this by sending laser light pulses through the cable. Any time a sound wave, seismic signal, and/or other pressure wave hits the underwater cable, the fiber slightly flexes (creating “strain modulations”). These small-scale movements cause anomalies that reflect and/or scatter some of the light back to the interrogator. Once a pulse of light has travelled to the end of the fiber and any reflections have travelled back to the interrogator, a subsequent laser pulse is introduced into the fiber without the risk of interference.
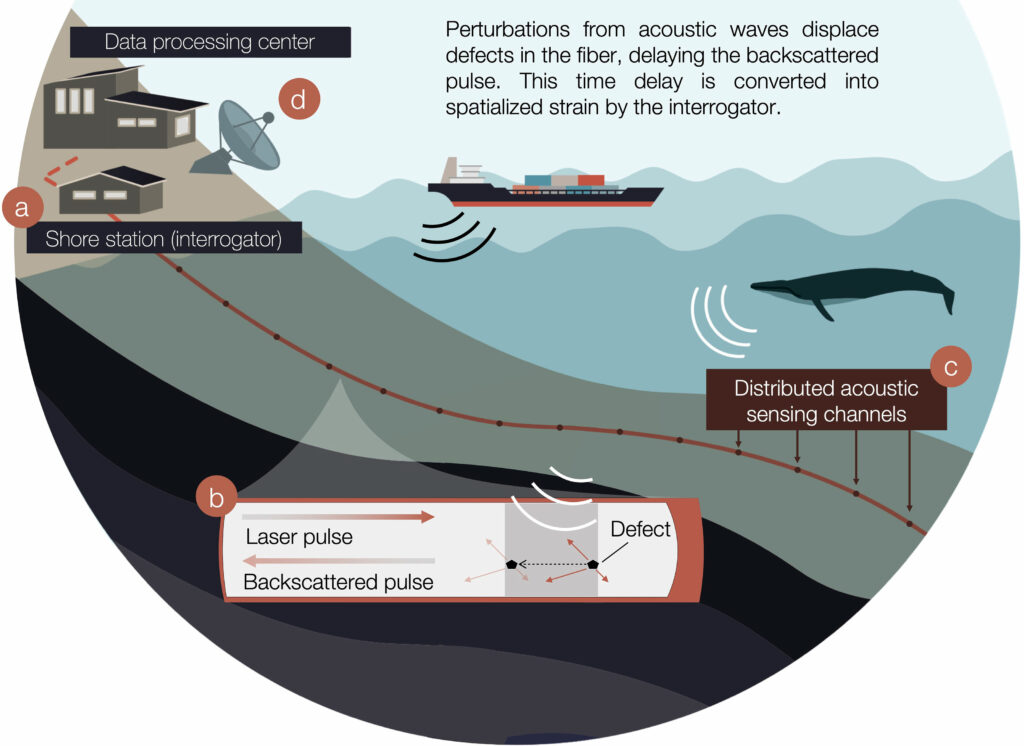
The interrogator records the reflected light pulses, calculating the time-differentiated backscattered response. This is done via consecutive sweeps at regularly spaced intervals along the entire length of the fiber. The time-differentiated phase changes are averaged and converted into waveforms, for each corresponding fiber section, and the full data set, sampled in both time and space, is streamed in near real-time to a land-based data processing center. Using advanced signal processing, the data is then translated into an acoustic signature, which can be used in the detection, identification, and/or localization of a potential acoustic source.
DAS has applications on land and underwater and has largely been used for geophysical applications such as monitoring earthquake signals, volcanic eruptions, and glacial movements. Researchers have since found the broad frequency band of DAS allows for the characterization and/or localization of different acoustic source types, such as distant storms, ocean swell, earthquakes, ships, and airguns. These signals may be received and classified simultaneously by the DAS system[2]Landrø, M., Bouffaut, L., Kriesell, H. J., Potter, J. R., Rørstadbotnen, R. A., Taweesintananon, K., Johansen, S. E., Brenne, J. K., Haukanes, A., Schjelderup, O., & Storvik, F. (2022). Sensing whales, storms, ships and earthquakes using an Arctic fibre optic cable. Scientific Reports, 12(1), 19226. https://doi.org/10.1038/s41598-022-23606-x.
DAS is now being explored as a passive acoustic monitoring tool. In July and August 2020, scientists tapped into an existing, unused, fiber-optic cable along Norway’s Svalbard archipelago to listen to and record whale vocalizations. This was the first time fiber optic cables had been used to detect whale songs[3]Bouffaut, L., Taweesintananon, K., Kriesell, H. J., Rørstadbotnen, R. A., Potter, J. R., Landrø, M., Johansen, S. E., Brenne, J. K., Haukanes, A., Schjelderup, O., & Storvik, F. (2022). Eavesdropping at the Speed of Light: Distributed Acoustic Sensing of Baleen Whales in the Arctic. Frontiers in Marine Science, 9, 901348. https://doi.org/10.3389/fmars.2022.901348[4]Landrø, M., Bouffaut, L., Kriesell, H. J., Potter, J. R., Rørstadbotnen, R. A., Taweesintananon, K., Johansen, S. E., Brenne, J. K., Haukanes, A., Schjelderup, O., & Storvik, F. (2022). Sensing whales, storms, ships and earthquakes using an Arctic fibre optic cable. Scientific Reports, 12(1), 19226. https://doi.org/10.1038/s41598-022-23606-x.The area, Isfjorden, is known as an Arctic summer feeding ground for baleen whales. Over 800 cetacean vocalizations were recorded during the 44-day study, with detections occurring out to at least 95 km along the cable[5]Bouffaut, L., Taweesintananon, K., Kriesell, H. J., Rørstadbotnen, R. A., Potter, J. R., Landrø, M., Johansen, S. E., Brenne, J. K., Haukanes, A., Schjelderup, O., & Storvik, F. (2022). Eavesdropping at the Speed of Light: Distributed Acoustic Sensing of Baleen Whales in the Arctic. Frontiers in Marine Science, 9, 901348. https://doi.org/10.3389/fmars.2022.901348.
In the summer of 2022, the researchers took their efforts a step further, working with two nearly parallel fiber-optic telecommunications cables in the same region off Svalbard. The scientists were able to track eight fin whales along a section of the cable for about five hours and estimate their position, effectively demonstrating how DAS could be used to detect, locate, and follow whales over a large (800 km2) area[6]Rørstadbotnen, R. A., Eidsvik, J., Bouffaut, L., Landrø, M., Potter, J., Taweesintananon, K., Johansen, S., Storevik, F., Jacobsen, J., Schjelderup, O., Wienecke, S., Johansen, T. A., Ruud, B. O., Wuestefeld, A., & Oye, V. (2023). Simultaneous tracking of multiple whales using two fiber-optic cables in the Arctic. Frontiers in Marine Science, 10, 1130898. https://doi.org/10.3389/fmars.2023.1130898. As the Arctic is rapidly changing, so is the timing and presence of whales in the region. Human activities, such as shipping, fishing, and tourism (e.g. cruise ships), are also increasing in the area. DAS could thus be used to help monitor whale presence and mitigate potential anthropogenic effects, such as ship strike.
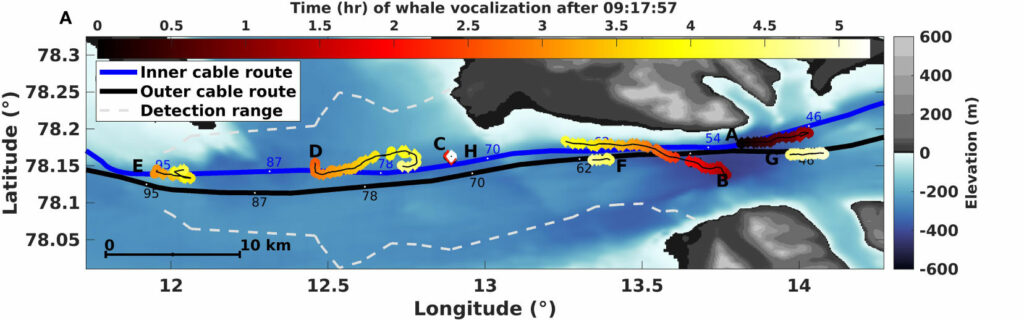
Other researchers have also used DAS technology for whale (and ship) detection. In November 2021, 4 days of DAS data were collected on two cables of the Ocean Observatories Initiative Regional Cabled Array extending offshore central Oregon. Numerous 20 Hz fin whale calls, northeast Pacific blue whale calls, and ship noises were recorded[7]Wilcock, W. S. D., Abadi, S., & Lipovsky, B. P. (2023). Distributed acoustic sensing recordings of low-frequency whale calls and ship noise offshore Central Oregon. JASA Express Letters, 3(2), 026002. https://doi.org/10.1121/10.0017104.
In addition to passive acoustic monitoring, DAS also has the capability to detect high frequency signals, such as those generated by vehicle thrusters, as well as underwater navigation, imaging, and communication systems. Thus, there is considerable potential for DAS and subsea surveillance and defense operations.
Despite its potential, it is important to note that DAS comes with a distinct set of challenges. DAS implementation is constrained by the relationship between the optical pulse intervals and the 2-way travel times along the physical length of the cable. The attenuation of the reflected light during its two-way travel will impact how far a signal can travel. Thus, a large-scale DAS array would require some type of amplification along its length. A tremendous amount of data is also generated by DAS. During their 44 days of recording, Boufatt et al. (2022) generated roughly 250 terabytes of data. Thus, there is a need for large scale data processing methods to scan, detect, and classify DAS outputs. Given the need for real time processing to detect potential signals of interest, the development of automated detection and classification methods is also key. Access to DAS interrogators is also limited, and the expense of the interrogator can cost more than $100,000. There are additional challenges with the fiber optic cables, as ownership is often private, requiring specific legal agreements for access. Lastly, many cables are equipped with signal boosters that can block an integrator’s laser pulses, reducing the usable length of a cable to the initial 50-70 km.
DAS offers a powerful, data-driven management tool that could be used to fill monitoring gaps in regions across the global ocean where instrumentation is difficult to implement (many telecommunication cables traverse remote, underwater regions that are otherwise challenging to access). Additional research and development are needed to better understand the instrument response of DAS and optimal monitoring strategies. However, as DAS technologies continue to develop, the world’s fiber-optic cable grid could be integrated with other remote-sensing systems, such as satellites, to create a global-scale, real-time, acoustic monitoring network.
DOSITS Links
- Science > What are common underwater sounds?
- Science > How does sound move? Reflection
- Science > How does sound move? Scattering
- Science > Advanced Topics > Signal Processing
- Animals > Effects > Anthropogenic Sound Sources > Commercial Vessel Traffic
- People > How is sound used to study underwater volcanoes?
- People > How is sound used to study undersea earthquakes?
- People > How is sound used to protect marine mammals?
- People > How is sound used in national defense?
- Technology Gallery > Ocean Observatories
- Audio Gallery > Blue Whale
- Audio Gallery > Fin Whale
- Audio Gallery > Earthquake
- Audio Gallery > Airgun
- Audio Gallery > Ship
- DOSITS Webinar Archive > Overview of Passive Acoustic Monitoring
Additional Resources
- EOS, Wiretapped Cables and the Songs of Whales
- Marine Technology News, Hearing the Light: DAS could Revolutionize Subsea Defense
- Lawrence Berkeley National Laboratory, U.S. Department of Energy, Using Fiber Optics to Advance Safe and Renewable Energy
- The New Yorker, Undersea Internet Cables Can Detect Earthquakes—and May Soon Warn of Tsunamis
- Polar Journal, Using fibre-optic cables to track whales
- Phys.org, July 2022, Eavesdropping on whales in the high Arctic
- Phys.org, May 2023, Using two fiber-optic cables to track whales as they cruise the Arctic
References
- Lindsey, N. J., Dawe, T. C., & Ajo-Franklin, J. B. (2019). Illuminating seafloor faults and ocean dynamics with dark fiber distributed acoustic sensing. Science, 366(6469), 1103–1107. https://doi.org/10.1126/science.aay5881
- Lior, I., Sladen, A., Rivet, D., Ampuero, J., Hello, Y., Becerril, C., Martins, H. F., Lamare, P., Jestin, C., Tsagkli, S., & Markou, C. (2021). On the Detection Capabilities of Underwater Distributed Acoustic Sensing. Journal of Geophysical Research: Solid Earth, 126(3), e2020JB020925. https://doi.org/10.1029/2020JB020925.
- Matsumoto, H., Araki, E., Kimura, T., Fujie, G., Shiraishi, K., Tonegawa, T., Obana, K., Arai, R., Kaiho, Y., Nakamura, Y., Yokobiki, T., Kodaira, S., Takahashi, N., Ellwood, R., Yartsev, V., & Karrenbach, M. (2021). Detection of hydroacoustic signals on a fiber-optic submarine cable. Scientific Reports, 11(1), 2797. https://doi.org/10.1038/s41598-021-82093-8.
- Rivet, D., de Cacqueray, B., Sladen, A., Roques, A., & Calbris, G. (2021). Preliminary assessment of ship detection and trajectory evaluation using distributed acoustic sensing on an optical fiber telecom cable. The Journal of the Acoustical Society of America, 149(4), 2615–2627. https://doi.org/10.1121/10.0004129.
- Sladen, A., Rivet, D., Ampuero, J. P., De Barros, L., Hello, Y., Calbris, G., & Lamare, P. (2019). Distributed sensing of earthquakes and ocean-solid Earth interactions on seafloor telecom cables. Nature Communications, 10(1), 5777. https://doi.org/10.1038/s41467-019-13793-z.
Cited References
⇡1 | Lindsey, N. J., & Martin, E. R. (2021). Fiber-Optic Seismology. Annual Review of Earth and Planetary Sciences, 49(1), 309–336. https://doi.org/10.1146/annurev-earth-072420-065213. |
---|---|
⇡2, ⇡4 | Landrø, M., Bouffaut, L., Kriesell, H. J., Potter, J. R., Rørstadbotnen, R. A., Taweesintananon, K., Johansen, S. E., Brenne, J. K., Haukanes, A., Schjelderup, O., & Storvik, F. (2022). Sensing whales, storms, ships and earthquakes using an Arctic fibre optic cable. Scientific Reports, 12(1), 19226. https://doi.org/10.1038/s41598-022-23606-x |
⇡3, ⇡5 | Bouffaut, L., Taweesintananon, K., Kriesell, H. J., Rørstadbotnen, R. A., Potter, J. R., Landrø, M., Johansen, S. E., Brenne, J. K., Haukanes, A., Schjelderup, O., & Storvik, F. (2022). Eavesdropping at the Speed of Light: Distributed Acoustic Sensing of Baleen Whales in the Arctic. Frontiers in Marine Science, 9, 901348. https://doi.org/10.3389/fmars.2022.901348 |
⇡6 | Rørstadbotnen, R. A., Eidsvik, J., Bouffaut, L., Landrø, M., Potter, J., Taweesintananon, K., Johansen, S., Storevik, F., Jacobsen, J., Schjelderup, O., Wienecke, S., Johansen, T. A., Ruud, B. O., Wuestefeld, A., & Oye, V. (2023). Simultaneous tracking of multiple whales using two fiber-optic cables in the Arctic. Frontiers in Marine Science, 10, 1130898. https://doi.org/10.3389/fmars.2023.1130898 |
⇡7 | Wilcock, W. S. D., Abadi, S., & Lipovsky, B. P. (2023). Distributed acoustic sensing recordings of low-frequency whale calls and ship noise offshore Central Oregon. JASA Express Letters, 3(2), 026002. https://doi.org/10.1121/10.0017104 |